For various purposes the knowledge concerning the spatial and in particular the vertical distribution of aerosols and cloud particles (and also of further trace material, as e.g. gaseous compounds) within in the atmosphere is essential. The strength and impact of sources for the atmospheric trace materials, the trace materials’ processing and altering, as well as the sinks of the atmospheric constituents are crucial key aspects to understand. Detailed knowledge concerning described aspects allows for investigating
- the dispersion of trace material, as e.g. pollutants, within the atmosphere,
- the trace materials’ atmospheric lifetime and persistence,
- the materials interaction with atmospheric water (e.g. to promote or to suppress clouds to form) or other chemical species, but also
- the trace material’s interaction with solar radiation (absorption, reflexion, diffraction),
all these topics if viewed in a comprehensive context also aim at gaining insights concerning the intensity and effectiveness of the trace materials’ impact on weather, climate and human health.
The Aerosol and Cloud Physics group at the Institute for Physics of the Atmosphere operates several instruments on board of various airborne platforms at different places in the world. The scientific question determines the aircraft to preferably use as respective platform features individual properties of maximum ceiling, cruising speed and/or operation range:
Aircraft used during research missions with our participation:
- M55 Geophysica
Missions: EUPLEX 2003 (Curtius et al., 2005), TROCCINOX 2005 (Borrmann, et al., 2010; Weigel et al., 2011), SCOUT-O3 2005 (de Reus, et al., 2009; Schumann, et al., 2017), SCOUT-AMMA 2006 (Frey et al. 2011; Frey et al. 2014), RECONCILE 2010 (von Hobe et al., 2013; Weigel et al., 2014), StratoClim 2016/2017 (Mahnke, 2018) - G 550 HALO
Missions: ML-CIRRUS 2014 (Voigt et al. 2016), ACCRIDICON-CHUVA 2014 (Wendisch et al. 2016) - Falcon-20
Missions: CONCERT - Learjet 35B
Missions: CIRRUS 2006, AIRTOSS, AIRTOSS-ICE 2013 (Klingebiel, et al. 2017) - ATR-42
Missions: EUCAARI 2008 (Crumeyrolle et al. 2010; Crumeyrolle et al. 2013) - POLAR-5/POLAR-6
Missions: VERDI 2012; RACEPACK 2014 (Klingebiel, et al. 2015) - Partenavia P68
Missions: MOSELDUNST
The instrument types that are in use by the Aerosol and Cloud Physics group can be distinguished correspondingly to their application:
-
One group of instruments (aiming at clouds’ microphysical properties, as e.g. number concentration, size distribution, effective radii, LWC/IWC, etc.) are usually mounted at underwing positions on respective aircraft. The optical measuring techniques used by these instruments are forward scattering of laser light, shadow cast imaging as well as digital holography. The instruments’ exposed mounting position and, in particular, an elevated flight speed cause the probed air to be compressed under sampling conditions which requires consideration if cloud elements’ microphysical properties are obtained from underwing cloud probe measurements as valid for ambient (undisturbed) conditions (cf. Weigel et al. 2016).
-
Aerosols in the submicron diameter size range are mainly focussed by using the second group of our instruments, generally deployed inside the aircraft’s cabin or within a dedicated payload bay interiorly of the aircraft’s fuselage. Such an instrument setup requires that the aerosol samples are taken from ambient conditions (outside the aircraft) by using a particularly designed inlet system, and the sample is transported through stainless steel aerosol lines across the aircraft’s hull towards the detecting instrument (cf. Weigel et al. 2009).
Our individual instruments provide measurement data over limited diameter size ranges, respectively. However, if the “visual fields” of the individual instruments are merged, this way up to two orders of magnitude, i.e. from ultrafine particles with 6 nm in diameter up to precipitating elements like rain droplets (or hail grains) with 6 mm diameter are covered by the ensemble of instruments used.
The work area of the Aerosol and Cloud Physics group covers diverse dues involving instrument development, modification, maintenance, characterization and operation during field missions. The obtained data are processed with state-of-the-art analysis techniques and the data products are evaluated and discussed in the scientific context within the Aerosol and Cloud Physics group and with a wide range of long-standing research partners at national and international institutions.
Atmospheric aerosols are the liquid or solid constituents suspended within a solvent, in this particular case, the air. As long as airborne, any solid or liquid particle (without bonded water) and also cloud particles, even precipitation elements (rain, drizzle, graupel, hail) are, per definition, implied in the expression aerosol. Atmospheric aerosols have various sources most of which are found at ground. Occasionally the release of aerosols has a natural origin (e.g. volcanic eruptions, desert dust storms, sea spray, boreal fires, meteoric ablation) but a significant fraction of particulate matter that is injected into the atmosphere is to ascribe to anthropogenic activities (e.g. emissions from industrial production and traffic, fossil fuel combustion, biomass burning, etc.).
The Aerosol & Cloud group mainly focusses at the aerosols present at up to altitudes of the upper troposphere/lower stratosphere (UT/LS). Of the entire middle atmosphere, a factor with one of the most immediate impacts on climate is the stratospheric aerosol layer, also known as Junge-layer. By scattering some parts of the incoming solar radiation back into space, the stratospheric aerosol has direct effects on the Earth’s energy balance. One example for this was the measurable and significant cooling as consequence of the Mount Pinatubo eruption in the year 1991. Besides its direct “shading effect”, stratospheric aerosol has another indirect effect by providing surfaces for heterogeneous reactions, which have an influence on the inventory of stratosphere’s chemical constituents, e.g. by promoting the quantity of active chlorine compounds that catalytically destroy ozone. The feedbacks of the ozone layer with climate are numerous (Scientific Assessment of Ozone Depletion: 2018). Recently, a comprehensive survey over the current state of knowledge concerning the stratospheric aerosol was provided by Kremser et al. (2016). Only two further of several other topics, the Aerosol & Cloud group is engaged in, may be introduced in the following:
At particular altitude layers, and if all prerequisites are available, aerosols can freshly form directly within the atmosphere. This source process, the aerosol production within the atmosphere, the so-called New Particle Formation (NPF) or gas-to-particle conversion, is one of the research fields that the Aerosol & Cloud group is dealing with. The image (left panel) shows two altitude levels over Brazil and Australia, at about 310 K and at about 360 K potential temperature, wherein excessive concentrations of ultrafine aerosol particles (diameter of 6-15 nm) were observed. At the same altitudes, the presence of refractory aerosol particles (as potential condensation surface) is relatively small (intermediate-right and outmost right panel). These very tiny particles in such huge amounts are suspected to have comparatively short atmospheric lifetimes (in the order of magnitude of hours up to very few days), thus are expected to have formed recently, prior to the observation.
Which precursor gases preferably form new particles, under which atmospheric conditions NPF is of particular intensity, and do these particles promote cloud formation within the UT or do they contribute to maintain the Junge-layer aerosol within the LS, these questions constitute a few of our research objectives..
Repeated measurements in the stratosphere over the Arctic winter pole revealed an increasing fraction (and number) of refractory aerosol particles (10 nm < Dp < 1 µm) with increasing altitude inside the polar vortex (cf. Image). It could be shown that the observed rise of particle mixing ratios is coupled with the air masses subsidence from very high (mesospheric) altitudes within the vortex, i.e. within the vortex, refractory aerosol material is transported downward from altitudes aloft the stratosphere. Two studies (Curtius et al., 2005 and Weigel et al., 2014) showed that high refractory aerosol mixing ratios correlate with low amounts of N2O (reddish data points). Air masses with low quantities of N2O are indicative for a particular air mass history: as N2O is photo-dissociated and oxidizes at very high altitudes within the atmosphere, where energy-rich radiation is capable to fraction N2O molecules. Thus, air masses enriched by refractory aerosol material and degraded by N2O must have been exposed to the energy-rich radiation for sufficient duration before the air masses subside within the vortex to stratospheric altitudes.
To investigate the nature of these refractory aerosol particles during several flights with the M55 Geophysica, aerosol samples were taken at stratospheric altitudes (> 410 K potential temperature) inside and outside the arctic winter vortex. These samples were analysed by means of Scanning Electron Microscopy (SEM) and Energy Dispersive X-ray (EDX) techniques by one of our partner institutes (Environmental Mineralogy, Inst. of Applied Geosciences, TU Darmstadt). Surprisingly, the variety of particle species found (embedded into a matrix of sulphuric and nitric acid - expected chemical aerosol species in the stratosphere) is huge. Some of the particles indicate previous high temperature exposure and the chemical (metallic) composition of others provide hints that meteoric ablation material (meteoric smoke) constitutes a fraction of the refractory aerosol material (Ebert et al. 2016). The origin of other particle are less unambiguous, and some indications are given that they may also origin from the ground (Schütze et al. 2017).
How the various aerosol species make up their way into the arctic stratosphere, how large is the contribution from meteoric ablation (smoke) material, and what may this aerosol impact once, the vortex dissolves in the Arctic spring period and releases the subsided aerosol material towards lower latitudes, these are further questions constituting another few of our research objectives.
Clouds are important for the local weather, therefore directly affecting everyone on a daily basis, but they also play an important role in the global climate. They are part of the hydrological cycle, transporting water to different places and producing precipitation, fuelling rivers and lakes. Especially persistent clouds affect the global radiation budget, since they reflect incoming solar light and trap outgoing heat – the reason for generally cooler nights and hotter days without clouds. Clouds can contain liquid water, ice or both, depending on the location and therefore the temperature: High clouds, such as cirrus, contain ice particles, whereas low clouds typically contain liquid water. Some clouds, such as cumulonimbus, span the entire troposphere and experience a variety of temperatures. In those clouds, one can find liquid water near the bottom and ice at higher altitudes. The exact composition of the cloud, i.e. which phase the water is in and how much water is present, but also how many and what kind of aerosol particles are there, determines key cloud processes such as formation, lifetime, precipitation formation and albedo (reflectivity). Hence, it is important to determine cloud properties to learn how clouds work, how they will behave, and what kind of impact clouds have.
One of the very particular cloud types are the polar stratospheric clouds (PSC). Their important role in stratospheric ozone-related chemistry distinguish into two major processes. The first is, they promote the heterogeneous activation of chlorine species. Secondly, due to their sedimentation PSC elements vertically redistribute water and nitric acid leading, the so-called process of dehydration and denitrification of the arctic stratosphere. Denitrification is assumed to elongate the period of efficient ozone depletion in the late spring during winters if the polar vortex remains stable and dissolves comparatively late. Current research still aims at ultimately quantifying the PSC’s contributions to polar ozone chemistry. Also not ultimately understood are the microphysical formation mechanisms that cause PSCs to develop. Different types of PSC particles are distinguished: most frequently occurring types of PSC particles are liquid: supercooled binary (SBS) or ternary solution (STS) droplets. SBS consists of sulfuric acid and water. STS additionally consists of nitric acid.
The image shows size distributions of detected PSC elements within the Arctic stratosphere compared to previous findings concerning PSC particles introduced as “NAT rocks”. The result of the comparison is published in Molleker et al. (2014) which was cited by the most recent Scientific Assessment of Ozone Depletion: 2018 from where the here shown graphic was adopted.
Holographic instruments are another type of underwing instruments that are designed to measure cloud elements. Contrary to the previously described instruments, which measure particles one at a time, holography is used to sample non-continuously larger local volumes of a few cubic centimetres and detect all droplets and ice crystals that are located within. The working mechanism for this includes a laser and a camera, where the laser beam is expanded and illuminates the sample volume. This sample volume is typically located between two forward pointing tips of the instrument, with as minimal influence to the airstream as possible. Any objects that happen to be within the sample volume will diffract the laser light and create a diffraction pattern that the camera records. Diffraction patterns from various objects will overlap and be recorded together. This resulting image is the hologram. From this, it is possible to reconstruct where the objects have been and what their shape was by reversing the light path. Since the laser emits one laser pulse at a time, the sample volume will be illuminated at different times and therefore different locations during the flight. Due to the large dimensions of the sample volume size distributions of cloud droplets and number concentrations can be derived from a local part of the cloud without the need to average over longer distances. Holography helps to determine the properties of neighbouring particles, such as spatial distributions or the phase, e.g. are ice crystals mixed randomly with liquid cloud droplets or not.
Both liquid cloud droplets as well as ice or snow crystals can be measured with holography, making the instruments useful for a variety of clouds that might contain both ice and liquid water. Furthermore, ice crystal habits and internal structure of the ice can be determined from the resulting images. Due to the pixel size of the used camera the minimum size that can be resolved is 6 microns, and the upper limit is typically determined by the concentrations – if there are too few particles, they are unlikely to be detected in the sample volume.
Our group has used a variety of holographic instruments on many different platforms. Following is a list of the missions including holographic instruments that are not only aircraft-borne, but also ground-based and laboratory-based measurements:
- CLACE2013 (Cloud and Aerosol Characterization Experiment, 2013): GipfelHolo (ground-based)
- RACEPAC (RAdiation and Cloud ExPeriment in the Arctic Circle, spring 2014) HALOHolo on Polar 6
- COSMICS (Cold-air Outbreak and sub-Millimetre Ice Cloud Study, spring 2015): HALOHolo on BAe-146 aircraft from FAAM
- CSET (Cloud System Evolution in the Trades, summer 2015): HOLODEC on NCAR G-V (HIAPER)
- ICE-D (Ice in Clouds – Dust, Summer 2015): HALOHolo on BAe-146 aircraft from FAAM
- Azores, summer 2017: HALOHolo on ACTOS
- ACE-ENA (Aerosol and Cloud Experiment in the Eastern North Atlantic, summer 2017 and winter 2018): HOLODEC on ARM G1.
- HIVIS (lab-based experiments 2015-2019)
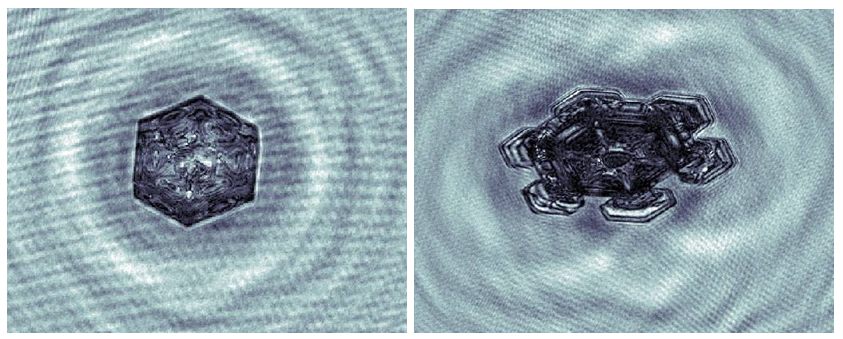
Ice crystals imaged with HOLODEC on the NCAR C-130 above Wyoming, USA
NCAR G-V (HIAPER)
Curtius, J.; R. Weigel, H.-J. Vössing, H. Wernli, A. Werner, C.-M. Volk, P. Konopka, M. Krebsbach, C. Schiller, A. Roiger, H. Schlager, V. Dreiling, S. Borrmann; Observation of meteoritic material and implications for Aerosol nucleation in the winter Arctic lower stratosphere derived from in situ particle measurements; Atmos. Chem. Phys., 5, 1-25, 2005.
Weigel, R., M. Hermann, J. Curtius, C. Voigt, S. Walter, T. Böttger, B. Lepukhov, G. Belyaev and S. Borrmann; Experimental characterization of the COndensation PArticle counting System for high altitude aircraft borne application; Atmos. Meas. Tech., 2, 243-258, 2009.
de Reus, M., S. Borrmann, A. J. Heymsfield, R. Weigel, C. Schiller, V. Mitev, W. Frey, D. Kunkel, A. Kürten, J. Curtius, N. M. Sitnikov, A. Ulanovsky, and F. Ravegnani; Evidence for ice particles in the tropical stratosphere from in-situ measurements; Atmos. Chem. Phys., 9, 6775-6792, 2009.
Borrmann, S., D. Kunkel, R. Weigel, A. Minikin, T. Deshler, J. C. Wilson, J. Curtius, G. N. Shur, G. V. Belyaev, K. S. Law, and F. Cairo, Aerosols in the tropical and subtropical UT/LS: in-situ measurements of submicron particle abundance and volatility, Atmos. Chem. Phys., 10, 5573-5592, 2010
Crumeyrolle, S., H. E. Manninen, K. Sellegri, G. Roberts, L. Gomes, M. Kulmala, R. Weigel, P. Laj, and A. Schwarzenboeck, New particle formation events measured on board the ATR-42 aircraft during the EUCAARI campaign, Atmos. Chem. Phys., 10, 6721-6735, 2010
Frey, W., S. Borrmann, D. Kunkel, R. Weigel, M. de Reus, H. Schlager, A. Roiger, C. Voigt, P. Hoor, J. Curtius, M. Krämer, C. Schiller, C. M. Volk, C. D. Homan, F. Fierli, G. Di Donfrancesco, A. Ulanovsky, F. Ravegnani, N. M. Sitnikov, S. Viciani, F. D'Amato, G. N. Shur, G. V. Belyaev, K. S. Law, and F. Cairo, In-situ measurements of tropical cloud properties in the West African monsoon: upper tropospheric ice clouds, mesoscale convective system outflow, and subvisual cirrus, Atmos. Chem. Phys., 11, 5569–5590, 2011..
Weigel, R., Borrmann, S., Kazil, J., Minikin, A., Stohl, A., Wilson, J. C., Reeves, J. M., Kunkel, D., de Reus, M., Frey, W., Lovejoy, E. R., Volk, C. M., Viciani, S., D'Amato, F., Schiller, C., Peter, T., Schlager, H., Cairo, F., Law, K. S., Shur, G. N., Belyaev, G. V., and Curtius, J.: In situ observations of new particle formation in the tropical upper troposphere: the role of clouds and the nucleation mechanism, Atmos. Chem. Phys., 11, 9983-10010, 10.5194/acp-11-9983-2011, 2011.
Crumeyrolle, S., R. Weigel, K. Sellegri, G. Roberts, L. Gomes, A. Stohl, P. Laj, G. Momboisse, T. Bourianne, V. Puygrenier, F. Burnet, F. Chosson, J. L. Brenguier, J. M. Etcheberry, P. Villani, J. M. Pichon, A. Schwarzenboeck, Airborne investigation of the aerosols–cloud interactions in the vicinity and within a marine stratocumulus over the North Sea during EUCAARI (2008), Atmospheric Environment, 81, 288 – 303, doi:10.1016/j.atmosenv.2013.08.035, 2013.
von Hobe, M., Bekki, S., Borrmann, S., Cairo, F., D'Amato, F., Di Donfrancesco, G., Dörnbrack, A., Ebersoldt, A., Ebert, M., Emde, C., Engel, I., Ern, M., Frey, W., Genco, S., Griessbach, S., Grooß, J. U., Gulde, T., Günther, G., Hösen, E., Hoffmann, L., Homonnai, V., Hoyle, C. R., Isaksen, I. S. A., Jackson, D. R., Jánosi, I. M., Jones, R. L., Kandler, K., Kalicinsky, C., Keil, A., Khaykin, S. M., Khosrawi, F., Kivi, R., Kuttippurath, J., Laube, J. C., Lefèvre, F., Lehmann, R., Ludmann, S., Luo, B. P., Marchand, M., Meyer, J., Mitev, V., Molleker, S., Müller, R., Oelhaf, H., Olschewski, F., Orsolini, Y., Peter, T., Pfeilsticker, K., Piesch, C., Pitts, M. C., Poole, L. R., Pope, F. D., Ravegnani, F., Rex, M., Riese, M., Röckmann, T., Rognerud, B., Roiger, A., Rolf, C., Santee, M. L., Scheibe, M., Schiller, C., Schlager, H., Siciliani de Cumis, M., Sitnikov, N., Søvde, O. A., Spang, R., Spelten, N., Stordal, F., Sumińska-Ebersoldt, O., Ulanovski, A., Ungermann, J., Viciani, S., Volk, C. M., vom Scheidt, M., von der Gathen, P., Walker, K., Wegner, T., Weigel, R., Weinbruch, S., Wetzel, G., Wienhold, F. G., Wohltmann, I., Woiwode, W., Young, I. A. K., Yushkov, V., Zobrist, B., and Stroh, F.: Reconciliation of essential process parameters for an enhanced predictability of Arctic stratospheric ozone loss and its climate interactions (RECONCILE): activities and results, Atmos. Chem. Phys., 13, 9233-9268, 10.5194/acp-13-9233-2013, 2013.
Weigel, R., Volk, C. M., Kandler, K., Hösen, E., Günther, G., Vogel, B., Grooß, J. U., Khaykin, S., Belyaev, G. V., and Borrmann, S.: Enhancements of the refractory submicron aerosol fraction in the Arctic polar vortex: feature or exception?, Atmos. Chem. Phys., 14, 12319-12342, 10.5194/acp-14-12319-2014, 2014.
Frey, W., Borrmann, S., Fierli, F., Weigel, R., Mitev, V., Matthey, R., Ravegnani, F., Sitnikov, N. M., Ulanovsky, A., and Cairo, F.: Tropical deep convective life cycle: Cb-anvil cloud microphysics from high-altitude aircraft observations, Atmos. Chem. Phys., 14, 13223-13240, 10.5194/acp-14-13223-2014, 2014.
Molleker, S., Borrmann, S., Schlager, H., Luo, B., Frey, W., Klingebiel, M., Weigel, R., Ebert, M., Mitev, V., Matthey, R., Woiwode, W., Oelhaf, H., Dörnbrack, A., Stratmann, G., Grooß, J. U., Günther, G., Vogel, B., Müller, R., Krämer, M., Meyer, J., and Cairo, F.: Microphysical properties of synoptic-scale polar stratospheric clouds: in situ measurements of unexpectedly large HNO3-containing particles in the Arctic vortex, Atmos. Chem. Phys., 14, 10785-10801, 10.5194/acp-14-10785-2014, 2014.
Klingebiel, M., de Lozar, A., Molleker, S., Weigel, R., Roth, A., Schmidt, L., Meyer, J., Ehrlich, A., Neuber, R., Wendisch, M., and Borrmann, S.: Arctic low-level boundary layer clouds: in situ measurements and simulations of mono- and bimodal supercooled droplet size distributions at the top layer of liquid phase clouds, Atmos. Chem. Phys., 15, 617-631, 10.5194/acp-15-617-2015, 2015.
Kremser, S., Thomason, L. W., von Hobe, M., Hermann, M., Deshler, T., Timmreck, C. Toohey, M., Stenke, A., Schwarz, J. P., Weigel, R., Fueglistaler, S., Prata, F. J., Vernier, J.-P., Schlager, H., Barnes, J. E., Antuña-Marrero, J.-C., Fairlie, D., Palm, M., Mahieu, E., Notholt, J., Rex, M., Bingen, C., Vanhellemont, F., Bourassa, A., Plane, J. M. C., Klocke, D., Carn, S. A., Clarisse, L., Trickl, T., Neely, R., James, A. D., Rieger, L., Wilson, J. C., Meland, B., Stratospheric aerosol - Observations, processes, and impact on climate; Review of Geophysics, 10.1002/2015RG000511, 2016.
Ebert, M., Weigel, R., Kandler, K., Günther, G., Molleker, S., Grooß, J. U., Vogel, B., Weinbruch, S., and Borrmann, S.: Chemical analysis of refractory stratospheric aerosol particles collected within the arctic vortex and inside polar stratospheric clouds, Atmos. Chem. Phys., 16, 8405-8421, 2016.
Manfred Wendisch; Ulrich Pöschl; Meinrat O. Andreae; Luiz A. L. Machado; Rachel Albrecht; Hans Schlager; Daniel Rosenfeld; Scot T. Martin; Ahmed Abdelmonem; Armin Afchine; Alessandro Araujo; Paulo Artaxo; Heinfried Aufmhoff; Henrique M. J. Barbosa; Stephan Borrmann; Ramon Braga; Bernhard Buchholz; Micael Amore Cecchini; Anja Costa; Joachim Curtius; Maximilian Dollner; Marcel Dorf; Volker Dreiling; Volker Ebert; Andre Ehrlich; Florian Ewald; Gilberto Fisch; Andreas Fix; Fabian Frank; Daniel Fuetterer; Christopher Heckl; Fabian Heidelberg; Tilman Hueneke; Evelin Jaekel; Emma Jaervinen; Tina Jurkat; Sandra Kanter; Udo Kaestner; Mareike Kenntner; Juergen Kesselmeier; Thomas Klimach; Matthias Knecht; Rebecca Kohl; Tobias Koelling; Martina Kraemer; Mira Krueger; Trismono Candra Krisna; Jost V. Lavric; Karla Longo; Christoph Mahnke; Antonio O. Manzi; Bernhard Mayer; Stephan Mertes; Andreas Minikin; Sergej Molleker; Steffen Muench; Bjoern Nillius; Klaus Pfeilsticker; Christopher Poehlker; Anke Roiger; Diana Rose; Dagmar Rosenow; Daniel Sauer; Martin Schnaiter; Johannes Schneider; Christiane Schulz; Rodrigo A. F. de Souza; Antonio Spanu; Paul Stock; Daniel Vila; Christiane Voigt; Adrian Walser; David Walter; Ralf Weigel; Bernadett Weinzierl; Frank Werner; Marcia A. Yamasoe; Helmut Ziereis; Tobias Zinner; Martin Zoeger., Introduction of the ACRIDICON-CHUVA campaign studying deep convective clouds and precipitation over Amazonia using the new German HALO research aircraft, DOI: http://dx.doi.org/10.1175/BAMS-D-14-00255.1, Bulletin of the American Meteorological Society, 2016.
Schledewitz, W., 2016: Holographische Messungen von Eis in Stratus-/ Stratocumuluswolken. University of Mainz, Diplom Thesis.
Christiane Voigt, Ulrich Schumann, Andreas Minikin, Ahmed Abdelmonem, Armin Afchine, Stephan Borrmann, Maxi Böttcher, Bernhard Buchholz, Luca Bugliaro, Anja Costa, Joachim Curtius, Maximilian Dollner, Andreas Dörnbrack, Volker Dreiling, Volker Ebert, Andre Ehrlich, Andreas Fix, Linda Forster, Fabian Frank, Daniel Fütterer, Kaspar Graf, Jens-Uwe Grooß, Silke Groß, Bernd Heinold, Tilman Hüneke, Emma Järvinen, Tina Jurkat, Stefan Kaufmann, Mareike Kenntner, Marcus Klingebiel, Thomas Klimach, Rebecca Kohl, Martina Krämer, Bernhard Mayer, Stephan Mertes, Andreas Minikin, Sergej Molleker, Andreas Petzold, Klaus Pfeilsticker, Markus Rapp, Marc Rautenhaus, Philipp Reuter, Christian Rolf, Diana Rose, Daniel Sauer, Andreas Schäfler, Romy Schlage, Hans Schlager, Martin Schnaiter, Johannes Schneider, Peter Spichtinger, Paul Stock, Ralf Weigel, Bernadett Weinzierl, Manfred Wendisch, Frank Werner , Heini Wernli, Martin Wirth, Andreas Zahn, Helmut Ziereis, Martin Zöger; ML-CIRRUS - The airborne experiment on natural cirrus and contrail cirrus with the new High Altitude LOng range research aircraft HALO, DOI: http://dx.doi.org/10.1175/BAMS-D-15-00213.1, Bulletin of the American Meteorological Society, 2016.
Weigel, R., Spichtinger, P., Mahnke, C., Klingebiel, M., Afchine, A., Petzold, A., Krämer, M., Costa, A., Molleker, S., Reutter, P., Szakáll, M., Port, M., Grulich, L., Jurkat, T., Minikin, A., and Borrmann, S.: Thermodynamic correction of particle concentrations measured by underwing probes on fast-flying aircraft, Atmos. Meas. Tech., 9, 5135-5162, https://doi.org/10.5194/amt-9-5135-2016, 2016.
Schumann, U., C. Kiemle, H. Schlager, R. Weigel, S. Borrmann, F. D'Amato, M. Krämer, R. Matthey, C. Voigt, M. Volk; Detection of contrails and convective cirrus above the tropical tropopause; Atmos. Chem. Phys., 17, 2311-2346, 2017.
Schütze, K., Wilson, J. C., Weinbruch, S., Benker, N., Ebert, M., Günther, G., Weigel, R., and Borrmann, S.: Sub-micrometer refractory carbonaceous particles in the polar stratosphere, Atmos. Chem. Phys., 17, 12475-12493, https://doi.org/10.5194/acp-17-12475-2017, 2017.
Schlenczek, O., 2017: Airborne and ground-based holographic measurement of hydrometeors in liquid-phase, mixed-phase and ice clouds. University of Mainz, PhD Thesis.
Glienke, S., A. Kostinski, J. Fugal, R. A. A. Shaw, S. Borrmann, and J. Stith, 2017: Cloud droplets to drizzle: Contribution of transition drops to microphysical and optical properties of marine stratocumulus clouds., Geophys. Res. Lett., 44, 8002–8010, doi:10.1002/2017GL074430.
Schlenczek, O., J. P. Fugal, G. Lloyd, K. N. Bower, T. W. Choularton, M. Flynn, J. Crosier, and S. Borrmann, 2017: Microphysical Properties of Ice Crystal Precipitation and Surface-Generated Ice Crystals in a High Alpine Environment in Switzerland. J. Appl. Meteorol. Climatol., 56, 433–453, doi:10.1175/JAMC-D-16-0060.1.
Mahnke, C.; Untersuchungen zu Wolkenbildung und Aerosolmikrophysik in der tropischen Troposphäre und UT/LS: Messtechnik und flugzeuggetragene in-situ Beobachtungen. Dissertation am Fachbereich Physik, Mathematik und Informatik der Johannes Gutenberg-Universität, Mainz, 2018
Larsen, M. L., R. A. Shaw, A. B. Kostinski, and S. Glienke, 2018: Fine-Scale Droplet Clustering in Atmospheric Clouds: 3D Radial Distribution Function from Airborne Digital Holography. Phys. Rev. Lett., 121, 204501, doi:10.1103/PhysRevLett.121.204501. link